How do neuronal processes degenerate?
The huge neuronal networks that run throughout our whole body are created when individual neurons build connections to other neurons, with the help of their processes. While neuronal processes are often degraded in the course of many neurological diseases, they are also degraded in healthy organisms. For example, adults have fewer neuronal connections than infants. That is because neurons initially build an excess of connections, some of which are specific and some of which are non-specific; later on, only the important and specific connections persist, whereas the others are degraded. Researchers name the degeneration of neuronal processes “pruning”. In my doctoral thesis, I analysed how the pruning process takes place and how it is regulated in the fruit fly.
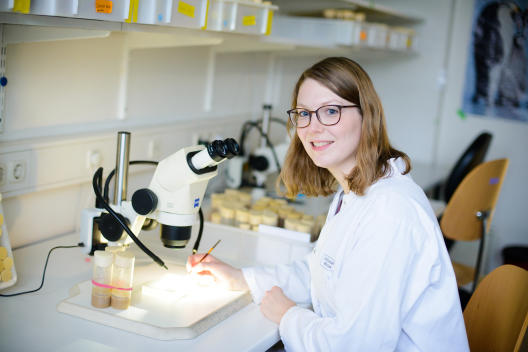
Do flies have neurons? Do they even have a brain?
When I tell friends about my research project, they often ask: “Do flies have neurons, or even a brain?” Fruit flies, also called Drosophila melanogaster, are only three millimeters long, but they actually contain a brain and a large neuronal network that runs through their body. They are more closely related to humans than some might believe. In flies as well as humans, neurons change their structure during development. When larvae pupate and transform into adult flies, its nerve cells have to adapt to the new body shape. To do so, neurons first degenerate their processes and later grow new processes. In Drosophila, we can easily analyse the degeneration of neuronal processes, the so-called pruning, in a short time since fruit flies only take ten days to develop.
During my doctoral thesis, I focused on a specific type of neuron in the skin of Drosophila. These neurons have a cell body, from which two different types of neuronal processes grow: many dendrites and an axon. The dendrites make contact with the skin and can sense pressure or heat. The axon transfers this information to other neurons in the brain. Compared to neurons in the fly brain, neurons in the skin are more easily accessible because they lie at the surface of the fly’s body. These skin neuron cells are not visible to the naked eye, since their cell bodies are only half the diameter of a human hair. The dendrites, which grow out of the cell body, are even smaller. To analyse these cells and their loss of dendrites, our flies are genetically modified so that the neurons in the skin produce a fluorescent dye. If this dye is illuminated with blue light, then the neurons reflect green light. This light can be captured with a fluorescence microscope, and a digital image of the neuron can be generated on a computer screen. If we follow the development of these neurons over several hours in a fly pupa, we can see that the dendrites are initially severed close to the cell body; then they disassemble into smaller pieces until finally only the cell body and an axon remain. Hence, neurons specifically remove their dendrites, but their cell body and axon remain intact. But how do these cells know that they should get rid of their dendrites and how does this process take place?
Reverse genetics helps us to understand the pruning process
To analyse processes in organisms in more detail, researchers use a technique called reverse genetics. Here, genes are switched off, and thereafter the effect on the process of interest is analysed. Because genes contain information for assembling proteins, which in turn carry out diverse functions within cells, one of my tasks during my doctoral thesis was to specifically switch off certain genes and then analyse whether the neural pruning process still occurred error-free. If the process was impaired or did not work at all, I could draw the conclusion – in a reverse manner, so to speak – that the specific gene and the resulting protein are important for the pruning process.
With the help of this method, I found that the protein PAR-1 is important for the pruning process. When the genetic sequence for PAR-1, which contains information about the PAR-1 protein, was altered, a pruning defect occurred: Neurons did not remove their dendrites.
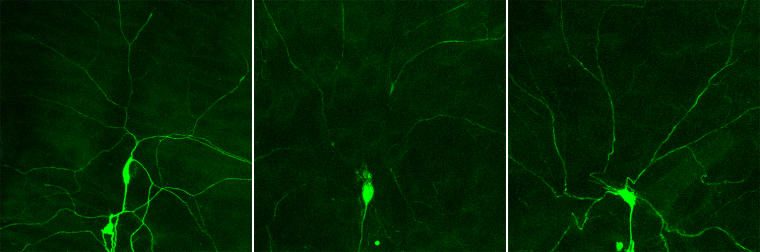
For a few years, it has been known that for dendrites to be successfully pruned, the cell’s skeleton (called the cytoskeleton) also needs to be disassembled. However, it is not yet known if there is a connection between the disassembly of the cytoskeleton and the function of the PAR-1 protein during pruning. Amongst other things, the cytoskeleton is made up from microtubules, which are tubular structures that stabilise cells – similar to the bones of our skeleton that stabilise our bodies. After I took a closer look at microtubules, it quickly became clear that neurons missing PAR-1 can no longer disassemble their microtubules.
How does the PAR-1 protein help disassemble microtubules? Proteins can serve as building blocks to stabilise structures within cells or the cell itself. However, some proteins also act as machines to carry out specific tasks within a cell. My literature search showed that PAR-1 is not a building block protein, but rather it acts like a machine: PAR-1 can activate or deactivate other proteins, such as the protein Tau. When Tau is active, it sits on microtubules and stabilises them. When it is inactive, Tau can no longer sit on the microtubules and stabilise them so the microtubules disassemble. From research on Alzheimer’s disease, it is known that the process of inactivating Tau is involved in the degeneration of neurons. In my work, I showed that in Drosophila, PAR-1 and Tau also interact during the development of a healthy organism and promote microtubule disassembly and dendrite removal. If only the PAR-1 gene is switched off, Tau stays active and stabilises microtubules – no pruning happens. However, if both PAR-1 and Tau are simultaneously switched off, dendrites can be removed normally. Thus, PAR-1 works by inactivating its target Tau in order to allow dendrite pruning.
Now we know what needs to happen in order for microtubules to disassemble and dendrites to be removed during pruning. But how does PAR-1 know when it is supposed to inactivate its target Tau? Well, that’s what research is like: One question is solved, but then another one arises.
Further information
- Publication: Herzmann S, Krumkamp R, Rode S, Kintrup C, Rumpf S. PAR-1 Promotes Microtubule Breakdown During Dendrite Pruning in Drosophila. EMBO J 2017; DOI: 10.15252/embj.201695890
- AG Dr. Sebastian Rumpf
Background information "Science in a way that everyone can understand"
This article is the result of a communication training for junior scientists. The participants learned techniques for writing an interesting, easily readable text. Subsequently, they wrote an article about their research that also non-experts should understand and translated it into English. The communication team of the Cells-in-Motion Cluster of Excellence initiated the project and supported the participants in individual coaching sessions. The English support office of the University of Münster helped to optimise the translations. The aims: Reflecting terms of content and language when editing their own research topic should benefit the participants in their communication with the public but also within the scientific community. They also gain experience in working with communication departments and photographers.
Further articles resulting from this project:
- How nerve cells communicate in the fear network – Guest contribution by Dr. Lena Goedecke, biologist in the research group of Prof. Hans-Christian Pape at the Cells-in-Motion Cluster of Excellence
- Developmental biology – My research about the coronary vasculature: Guest contribution by Dr. Guillermo Luxán, biologist in the research group of Prof. Ralf Adams at the Cells-in-Motion Cluster of Excellence
- How the cells’ environment affects their migration: Guest article by Sargon Groß-Thebing, PhD student in the group of Prof. Erez Raz at the Cells-in-Motion Cluster of Excellence