Ultrarelativistic Heavy Ion Collisions

Protons and neutrons, the constituents of the atomic nucleus, are composed of quarks, bound together by gluons, the carriers of the strong force.
Quarks have not been observed as free particles; their confinement in protons and neutrons and other particles is apparently a law, and at the same time a mystery, of nature. According to the standard model of the universe, the Big Bang model, up until about 10 microseconds in the age of the early universe space was filled by freely roaming quarks and gluons, the so-called quark-gluon plasma. In a phase transiton, that state of matter gave birth to protons and neutrons as basic building blocks of our known matter. Today the temperature and density of this phase transition can be computed quite precisely in calculations if Quantum Chromodynamics (QCD), the theory of strong interaction.
An experimental program at the Large Hadron Collider (LHC) at CERN and and at other facilities is ongoing to study the properties of quark-gluon matter produced for a very short time in ultrarelativistic heavy-ion collisions.
Our research group is continuing the work of the group of Prof. Dr. R. Santo, who participated in the first experiments of this kind at the SPS accelerator at CERN. The measurement of direct photons in these experiments yielded a significant insight into the properties of hot and dense quark-gluon matter.
At the Relativistic Heavy-Ion Collider (RHIC) at Brookhaven National Laboratory, USA, collisions an order of magnitude more energetic than those at the CERN SPS were investigated since the start of the experimental program in year 2000. Our research group has participated in the PHENIX experiment at RHIC, under the leadership of Prof. Santo and later of Prof. Dr. J.P. Wessels. We have built and were in charge of a lead glass array consisting of about 10000 individual detector modules. One of the most important outcomes of the first years was the measurement of jet quenching at high transverse momentum, a key result for the studies of deconfined quark-gluon matter.
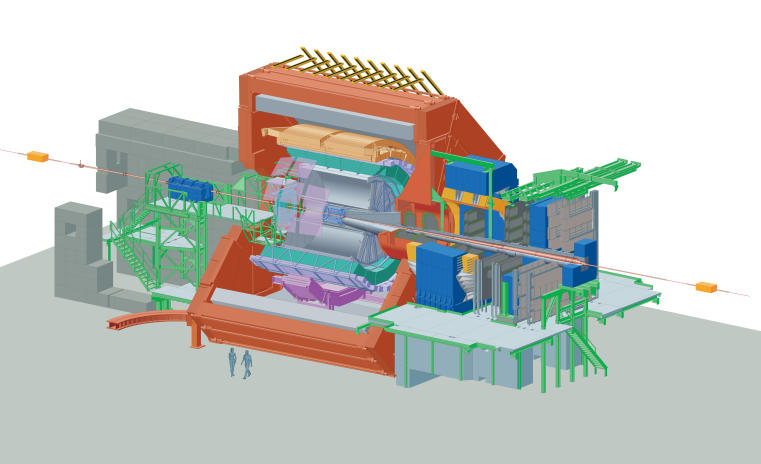
Another huge increase in the collision energy was achieved with the LHC at CERN. The machine started operation in 2009 and collided lead beams for the first time in 2010. We are long-time participants in the dedicated heavy ion experiment ALICE. One of the central detectors of ALICE is a large Transition Radiation Detector (TRD), which we built and operate, in close collaboration with other European institutes and universities.
Transition radiation X-rays are produced by the passage of highly relativistic charged particles through layers of material with different indices of refraction. Transition radiation detectors are unique tools for separating high energy electrons and positrons from charged pions and other hadrons. In addition, the detector provides a second level trigger for electrons, heavy fragments and jets.
Our group was involved in the detector development, particularly in the design, test and constructing the radiators. At IKP we assembled and tested 17 of the 18 TRD supermodules, each containing 30 individual detectors. We were also involved in the development of a software framework for simulations and data analysis on the Grid. Our physics interests and activities concern various aspect of jet production as well as photons and neutral mesons and quarkonia. We study those in proton-proton, proton-lead and lead-lead collisions in ALICE and perform also phenomenology analysis of data, in collaboration with colleagues at WWU Münster and at other universities (see, for instance, this press release).
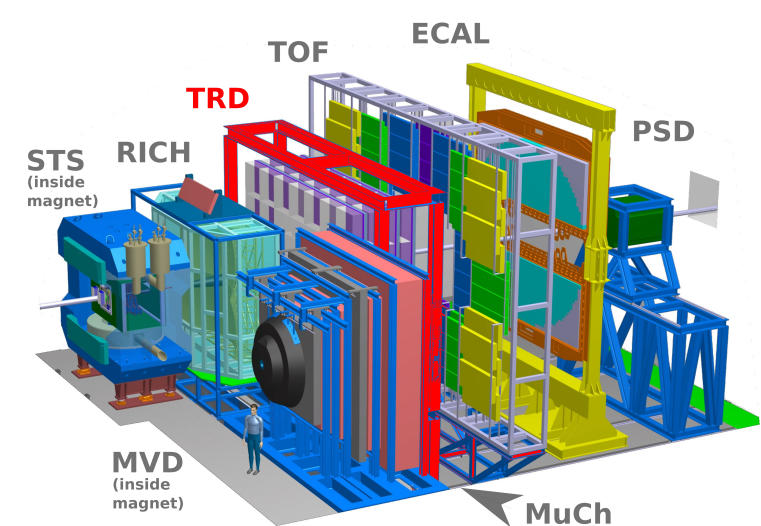
Our second line of research is devoted to the Compressed Baryonic Matter (CBM) experiment at the Facility for Antiproton and Ion Research (FAIR), currently under construction at GSI Darmstadt and expected to come in operation in 2028. The lower energy collisions that will be investigated with CBM at FAIR correspond rather closely to regimes encountered in neutron star collisions, where hot and dense nuclear matter is produced. The CBM experiment is expected to also provide definite proof whether deconfined matter is produced in this regime or not, via a multitude of observables such as the production of hypernuclei or dileptons.
The challenge of the CBM experiment are high collision rates in a fixed-target mode, which will be recorded in a continuous mode and selected online. In programs funded by the EU, our group has participated in the development of novel gas detectors with high rate capability. Aside from their use in nuclear and particle physics experiments these detectors also bear an enormous potential for use in medical imaging with X-rays.
We are much involved in beam tests of detector prototypes and are also currently constructing at IKP part of the final detectors; we are also responsible of their later integration in the CBM setup.