Evolution of the Earth
Magma Ocean
During Earth’s formation (about 4.5 billion years ago), giant impacts of mars-sized bodies onto the early Earth caused large melt layers (magma oceans) on Earth. Some of these have reached several hundred kilometers depth and covered the whole Earth. During the magma ocean period, the Earth rotated much faster than today, completing one turn in 5 hours as opposed to 24 hours today.
We investigate the influence of planetary rotation on the dynamics of rock crystals in a magma ocean by means of computer experiments in a rotating spherical shell. Our results suggest that planetary rotation may lead to the formation of large-scale chemical structures in the deep Earth (Maas & Hansen, 2019). These anomalies are still observed at present by seismology.
After Earth's cooling the magma ocean is solidified which could lead to the presence of rock layers with different compositions in the mantle. This which would have a major impact on further convection in the mantle.
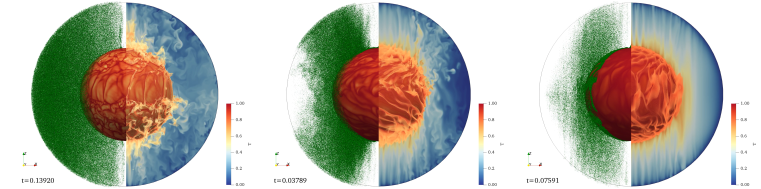
Mantle Convection
The core-mantle boundary (CMB) represents the lower boundary layer of the Earth's mantle and is structurally very complex with thermal plumes, thermochemical piles (LLSVPs) and subducted surface plates.
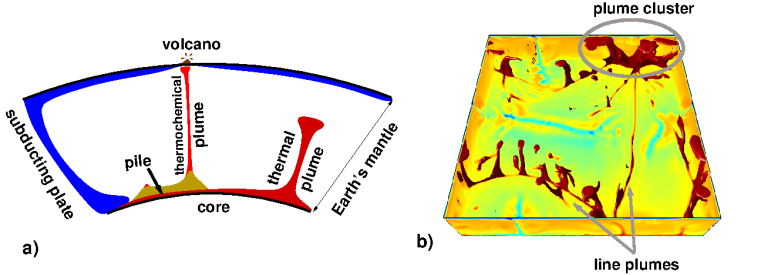
Seismologically observed features at the core-mantle boundary (CMB) possibly have a higher density than their surroundings. These structures could have formed from a dense layer that stems from the magma ocean period and/or, according to geochemical analyses, could be an indication of some core material being present within the mantle.
We therefore investigate core-mantle interaction as a possible origin. Our simulations show that the infiltration of iron-rich material into the mantle is coupled to the convective mantle flow. Long-lived accumulations (piles) of dense material form (Stein and Hansen, 2023).
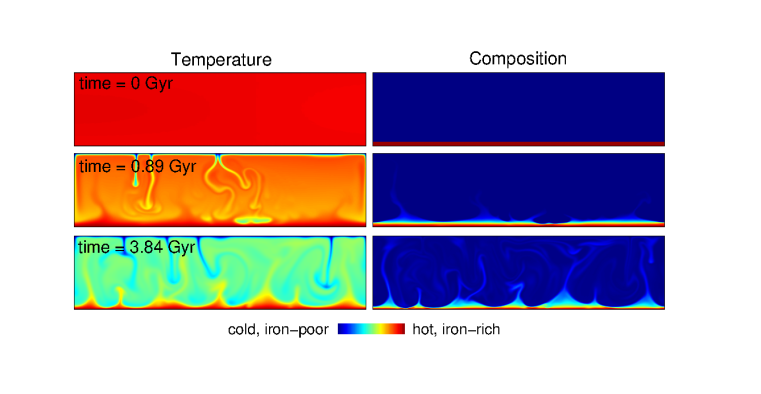
Plate Tectonics
Plate tectonics represents the expression of mantle convection at the Earth's surface. In this process, the Earth's plates move at a rate of a few centimetres per year, are generated at mid-ocean ridges, and sink back into the Earth's interior at subduction zones. A variety of geological observations, such as volcanic eruptions and earthquakes, can be seen at the plate boundaries.
We use computer models to study how plate tectonics evolved under the influence of dense structures in the Earth's deep mantle. Such structures of dense, iron-rich material have a stabilising influence on mantle flow (Trim et al., 2014).
For instance, we found that the presence of dense material delays the tectonic evolution. A mobile state similar to modern-style plate tectonics is reached later. It develops after passing through different stages: beginning from an immobile surface lid over episodic surface mobilisation (Kreielkamp et al., 2022).
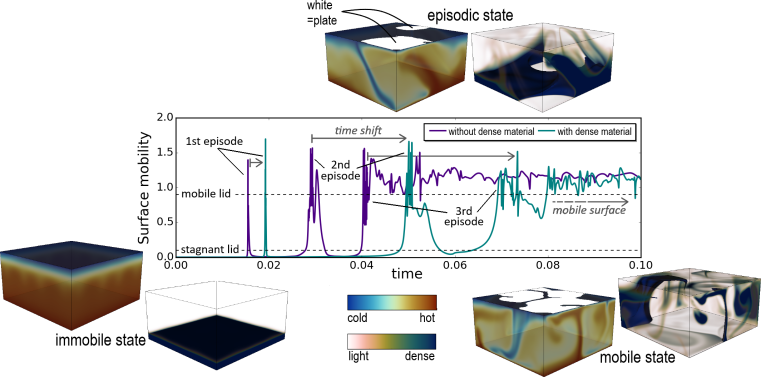